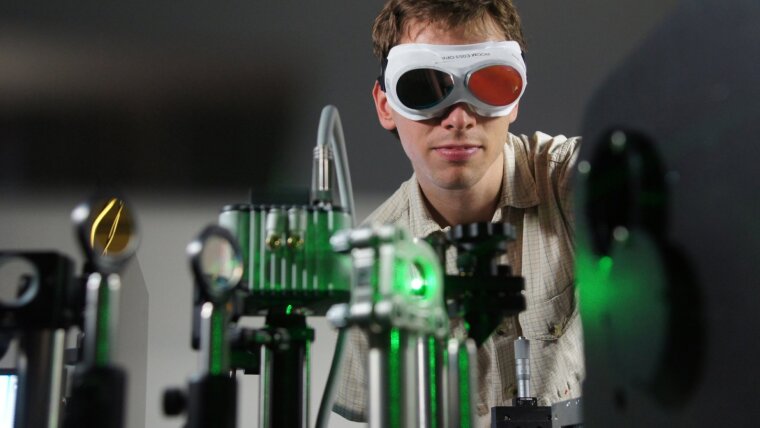
Obtaining a Master's degree at the Abbe School of Photonics means to immerse deeply in the rich and stimulating research environment of our Abbe Center of Photonics. Thus, our Master's degree students obtain significant hands-on experience by practical modules in state-of-the-art photonics laboratories. By choosing the respective elective modules, our students can specialize at least in the one out of five key education areas already during their Master's degree studies.
Fiber laser amplifying system.
Image: Jan-Peter Kasper (University of Jena)Our key education area Laser and strong field physics covers activities ranging from the development of lasers able to create extreme intensities (>1020 W/cm2) or ultra-high average powers above the kW barrier. They include the control of laser radiation at ultrafast time scales as well as theoretical and experimental studies on light-matter interactions under extreme conditions and in novel structures. Our education program is based on research which, for example, result in the invention and implementation of innovative light sources. These novel sources of light are at the same time highly integrated by the use of novel multifunctional components, as they offer some truly remarkable parameters along with flexible properties tailored to applications‘ needs. Beyond light sources, we are also exploring fundamentals of light-matter interaction. Specializing in Laser and strong field physics allows our graduates to develop new schemes for spectroscopic measurements, material processing, medical treatment, remote sensing, as well as to research at the forefront of the recent findings on the spatio-temporal light dynamics and extreme light states.
Spectroscopic characterization of an artificial nanooptical metamaterail.
Image: Jan-Peter Kasper (University of Jena)State-of-the-art nanofabrication technology allow for the realization of optical structures with sub-wavelength and therefore sub-micron dimensions. These structures can be either tiny photonic components, such as, e.g. waveguide bends, apertures, microdiscs, and nanoantennas, or they can exhibit periodic arrangements as e.g. diffraction gratings, photonic fibers, artificial crystals and metamaterials. In close collaboration between theory, technology, and experiments, fundamental effects of nanooptics are examined and explored by our scientists and students specializing in Nanooptics. Examples of recent research activities of our local scientists targeted the strong coupling of plasmonic nanoantennas to quantum systems, the light-induced self-organization of photonic nanostructures, the synthesis of nano-scaled multiphoton light sources, or the generation and control of diffractionless plasmonic beams, just to mention a few.
Frequency doubling in a nonlinear optical crystal.
Image: Jan-Peter Kasper (University of Jena)From a global perspective, Biophotonics has already provided major innovations for biomedical research and clinical routine and developed into a coherent scientific discipline of high societal and economic importance. In biomedical research, the recent development of ultrahigh resolution microscopy is providing novel insight into the nanoworld. Students specializing in this area are able to study processes in living cells and the development of diseases in much greater detail. Spectroscopic and multimodal imaging methods contribute complementary information on cell function and metabolism. These findings help in developing targeted therapies that treat diseases right at their origin – possibly even before manifest symptoms appear. Our biophotonics research explores methods that provide deeper insights into complex biological samples of different size, starting from organs via tissue sections, cells, viruses, down to DNA and RNA. Also in clinical routines, photonic technology enable early and sensitive and accurate recognition of diseases, as well as their gentle treatment. For example, fluorescent imaging has become an important method in the in vivo detection of cancer and can guide the surgeon with greater precision while operating. Current research aims to refine these techniques and detect tumors as small as one millimeter in diameter. Marker-free imaging methods, like Raman and near-infrared spectroscopy, are also developing towards in-vivo application and will provide even more detailed diagnostic information. The research performed by our scientists utilizes and develops these methods according to the needs of pathology, oncology, and sepsis.
Optical lithography of CMOS components.
Image: Jenoptik AGConsequently, it is not only due to the local history of Ernst Abbe, Otto Schott, and Carl Zeiss that Jena is one of the strongest places for the design and realization of modern optical systems. Our key education area Optical instrumentation and systems involves enabling fields such as lens design, aberration theory, system metrology, and performance evaluation as well as novel approaches for electromagnetic wave-based rigorous modeling. The latter is necessary when the applications require pushing the limits of classical optics, i.e. when including diffractive elements in the system. Similarly, our excellent research infrastructure and expertise allows our students already before graduation to get involved with optical instruments based e.g. on lithographically defined diffractive elements, microstructured fibers produced by sophisticated drawing technology, laser written waveguides, free-form surfaces, extreme thin-film technology and optoelectronic signal processing. The resulting optical systems find regular use in such extraordinary applications as real time 3D shape recognition, astronomic instruments for space missions, or in next-generation instruments for gravitational wave detection.
Entangled photons quantum setup.
Illustration: Gilaberte et al., "Video rate imaging with undetected photons," Laser Phot. Rev. 15, 2000327 (2021).Also at our Abbe School of Photonics, Quantum photonics has become a key education area and a major, fascinating field of research. Compared to other international quantum photonics hotspots, Jena's individual strength lies in our demonstrated ability to fuse multiple expertises to integrate available enabling technology into a combined research effort to open up new platforms and integrated systems exploiting quantum technology. One recent example is the generation of non-classical states of light, e.g. photon pairs, by spontaneous nonlinear processes in nonlinear photonic systems ranging from bulk crystals over different waveguide structures to nanostructured or atomically thin surfaces. This understanding can be used to tailor the properties of the generated two-photon quantum states, like spectrum, spatial distribution, and entanglement, to meet the demands of specific applications. Another very active research focus, open for specialized graduates in Quantum photonics, is the development of novel quantum light sources for applications in quantum communication and sensing, efficient processing and detection schemes for high-dimensional quantum information, as well as scalable methods for the transmission of quantum states over long distances.