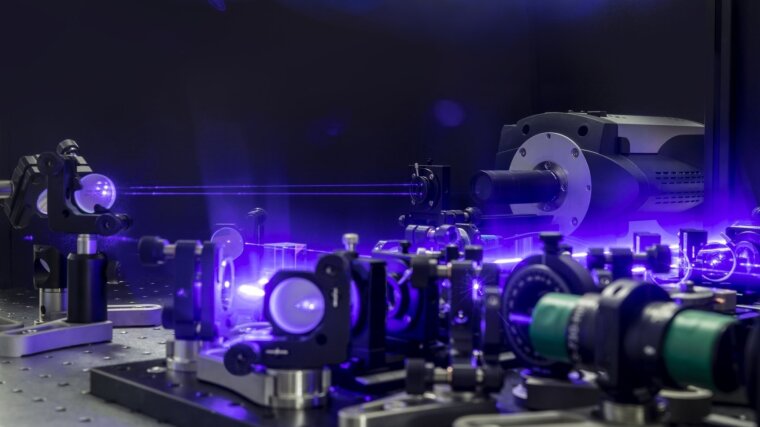
-
Advanced Quantum Theory (Bernuzzi/Gies)
Content
- Many particle systems, identical particles, non-interacting particles, Thomas-Fermi and Hartree-Fock approximations
- Addition of angular momenta, Clebsch-Gordan coefficients, selection rules
- Time-dependent perturbation theory, Fermis golden rule
- Scattering theory, potential scattering, partial waves, scattering of identical particles
- Introduction to relativistic quantum mechanics, Poincare transformations, Klein Gordon and Dirac equations, minimal coupling, non-relativistic approximation
- Relativistic hydrogen atom, fine structure
- Path integrals
Intended Learning Outcomes
Knowledge of relevant facts about advanced quantum mechanics which are necessary for an understanding of quantum phenomena and their relevance in all areas of modern physics. Ability to apply methods for describing and modeling nonrelativistic and relativistic quantum systems. Ability to solve demanding problems and deal with complex physical systems.
-
Fundamentals of Modern Optics (Pertsch)
Content
- Basic concepts of wave optics
- Dielectric function to describe light-matter interaction
- Propagation of beams and pulses
- Diffraction theory
- Elements of Fourier optics
- Polarization of light
- Light in structured media
- Optics in crystals
Intended Learning Outcomes
The course covers the fundamentals of modern optics which are necessary for the understanding of optical phenomena in modern science and technology. The students will acquire a thorough knowledge of the most important concepts of modern optics. At the same time, the importance and beauty of optics in nature and in technology will be taught. This will enable students to follow more specialized courses in photonics.
Recommended Reading
- B.E.A. Saleh and M.C. Teich, Fundamentals of Photonics, Wiley.
- H. Lipson, D.S. Tannhauser, S.G. Lipson, ”Optical Physics”, Cambridge.
- E. Hecht and A. Zajac, ”Optics”, Addison-Wesley Longman.
- F.L. Pedrotti, L.S. Pedrotti, L.M. Pedrotti, Introduction to Optics, Pearson.
- G. Brooker, Modern Classical Optics, Oxford.
-
General Relativity (Brügmann)
Content
- Fundamentals of general relativity
- Einstein field equations
- Newtonian approximation
- Gravitational waves
- Black holes
- Cosmology and the big bang
Intended Learning Outcomes
Obtain knowledge of relativistic gravitational physics. Develop problem solving skills for astrophysical problems in the regime of high velocities and strong gravitational fields.
-
Introduction to Quantum Physics (Setzpfandt)
Content
- Relevant core concepts of atomic and solid-state physics
- Basics of light-matter-interaction
- Basics of superconductivity
- Basics of quantum theory
- Quantum harmonic oscillator
- Perturbation theory
- Pictures of quantum mechanics
Intended Learning Outcomes
Understanding of basic concepts and methods for the description of physical systems within the framework of quantum theory. Ability to independently solve simple tasks in the area of quantum physics.
-
Physical Chemistry (Schmitt)
Content
- Equilibrium thermodynamics: Properties of gases, first and second law of thermodynamics, chemical equilibrium, equilibrium electrochemistry
- Transport phenomena: molecular motion in gases and liquids, diffusion, transport across biological membranes
- Chemical reactions: chemical kinetics, rate laws, temperature dependence of reaction rates, relaxation methods, kinetics of complex reactions
- Basics of quantum mechanics: wavefunctions and operators, particle in a box, harmonic oscillator, particle on a sphere, rigid rotator
- Approximations: variational principle, Born-Oppenheimer approximation, linear combination of atomic orbitals (LCAO) method, Hartree-Fock, density functional theory (DFT)
Intended Learning Outcomes
Understandig of the fundamentals of physical chemistry. Knowledge in equilibrium thermodynamics, chemical kinetics and basic molecular quantum mechanics.
-
Fundamentals of Quantum Information (Steinlechner/Setzpfandt/Gärttner)
Content
Concepts of quantum information processing
- Introduction to fundamental concepts and the basic formalism
- Entanglement
- Application examples
- Entanglement characterization
Hardware for quantum information processing
- Brief review of key physical concepts and applications
- Basic hardware requirements for information processing
Intended Learning Outcomes
Understanding of fundamental properties of quantum states, their applications and how to characterize them. Knowledge about basic hardware requirements for quantum information processing and example implementations.
-
Quantum Laboratory (Setzpfandt)
Content
Practical training in experimental quantum technology. Topics cover a broad range from quantum-state generation and characterization, the demonstration of fundamental quantum effects to applications in communication and metrology.
Intended Learning Outcomes
- Introduction to experimental techniques in quantum technology.
- Planning and preparation of a scientific measuring task.
- Carrying out scientific lab in optics together with a research team.
- Preparation of a scientific report.
-
Advanced Quantum Information (Steinlechner/Setzpfandt/Gärttner)
Content
Hardware for quantum information processing
- Superconducting qubits, gates, control, manipulation, readout
- Light-matter interaction
- Semiconductor qubits (quantum dots, defects)
- Atoms / quantum gases
- Foundations of quantum sensing (sensitivity, noise, standard quantum limit)
- Optomechanics
Concepts of quantum information processing
- Decoherence
- Quantum error correction
- Many-body entanglement
Intended Learning Outcomes
Knowledge of all eminent concepts for implementing quantum-information systems. Understanding advanced concepts that enable treatment of non-ideal quantum systems.
-
Advanced Seminar Gravitational and Quantum Physics (Brügmann/Gies)
Content
- Systematic development of specialized knowledge in the fields of gravitation theory and quantum theory
- Presentation and discussion of current problems of gravitation theory and quantum theory
Intended Learning Outcomes
- Familarisation with a specific topic in gravitation or quantum theory
- Independent discovery and evaluation of scientific literature
- Presentation of scientific facts in form of a talk
- In-depth knowledge in the fields of gravitation theory and quantum theory
-
Computational Physics II (Brügmann)
Content
- Introduction to Unix and higher-level programming languages (e.g.: C/C++, Fortran)
- Numerical solution of partial differential equations
- Monte Carlo method
- Molecular dynamics methods
- Minimization problems
Intended Learning Outcomes
Teaching the basic algorithms and practical skills for the numerical solution of complex physical problems and visualization of large amounts of data.
-
Electronic Structure Theory (Peschel)
Content
- Introduction to the many-body problem
- Wavefunction-based approaches for electronic structure
- Density functional theory
- Electronic excitations: beyond density functional theory
Intended Learning Outcomes
Electronic structure theory is a successful and ever-growing field, shared by theoretical physics and theoretical chemistry, that takes advantage from the increasing availability of high-performance computers. Starting only from the knowledge of the types of atoms that constitute a material (molecule, solid, nanostructure,..) we will learn how to determine without further experimental input, i.e. using only the laws of quantum physics, its structural and electronic properties.The lecture will initiate the students to the state-of-the-art theoretical and computational approaches used for electronic structure calculations. In the practical classes the students will learn through tutorials to use different software for electronic structure simulations. During the last month they will realize a small independent scientific project.
-
Molecular Quantum Mechanics / Quantum Chemistry I (Gräfe/Croy)
Content
In lecture and tutorial, students are taught basics and concepts describing the dynamics of (open) quantum systems (wave packets, density matrix, quantum master equations). Furthermore, aspects of multi-particle physics of molecules are covered, i.e. e.g. multi-electron wave functions, the Hartree-Fock approximation and the role of basis sets.
Intended Learning Outcomes
Become familiar with the fundamentals of open quantum systems and "ab initio" methods for performing quantum chemical calculations with respect to molecular and nanoscale systems.
-
Nanomaterials and Nanotechnology (Ronning - in German)
Content
- Dimension effects
- Quantisation of electrons
- Single-electron transistor
- Synthesis of nanomaterials
- Characterization of nanomaterials
- Material systems: carbon nanotubes, graphene, magnetic nanomaterials, bionanomaterials
- Application and technology of nanomaterials
Intended Learning Outcomes
In-depth knowledge in the field of solid-state physics.
-
Semiconductor Nanomaterials (Staude)
Content
- Review of fundamentals of semiconductors
- Optical and optoelectronic properties of semiconductors
- Effects of quantum confinement
- Photonic effects in semiconductor nanomaterials
- Physical implementations of semiconductor nanomaterials, including epitaxial structures, semiconductor quantum dots and quantum wires
- Advanced topics of current research, including 2D semiconductors and hybrid nanosystems
Intended Learning Outcomes
This course aims to convey a fundamental understanding of the physics governing the optical and optoelectronic properties of semiconductor nanomaterials. First, the fundamental optical and optoelectronic properties of bulk semiconductors are reviewed, deepening and extending previously obtained knowledge in condensed matter physics. The students will then learn about the effects of quantum confinement in semiconductor systems in one, two or three spatial dimensions, as well as about photonic effects in nanostructured semiconductors. Finally, several relevant examples of semiconductor nanomaterial systems and their applications in photonics are discussed in detail. After successful completion of the course, the students should be capable of understanding present research directions and of solving basic problems within this field of research.
-
Solid State Optics I (H. Schmidt)
Content
- Electronic, dielectric, and optical properties of solids
- Mueller matrix polarimetry
- Electrooptics and magnetooptics
- Photodetectors and optical systems
- Quantum optics and quantum technology
Intended Learning Outcomes
The course covers basic and advanced topics of solid state optics, with a special focus on the relation between electronic and optical properties. An effort is made to treat electro- and magnetooptical effects and quantum optical effects as rigorous as possible through the Mueller matrix approach and through quantum mechanical approaches, respectively.
-
Quantum Computing (Eilenberger/Steinlechner/Pertsch)
Content
- Basic introduction to algorithms and computing
- The Qubit and entanglement thereof
- Basics of quantum algorithms
- Advanced quantum algorithms
- Implementation of QuBits and quantum computers
- Hands-on circuits
Intended Learning Outcomes
After active participation in the course, the students will be familiar with the basic concepts of quantum computation and the implementation of quantum algorithms. They will be able to apply their knowledge in the assessment and creation of quantum algorithms and the development of quantum information systems. The intended learning outcome is to introduce the students to the basic usage of quantum bits for information processing. To provide further insight, the course will expand this concept on multipartite systems and introduce the concept of entanglement. In a further step we shall see how individual quantum operations tie together to create algorithms. Important algorithms, such as the quantum Fourier transformation, the algorithms of Shor and Grover will be discussed. To relate the abstract knowledge on quantum algorithms to practical applications, real-world implementations of quantum computers will be discussed.
-
Quantum Communication (Steinlechner/Eilenberger)
Content
- Basic introduction to quantum optics
- Quantum light sources
- Encoding, transmission and detection of information with quantum light
- Quantum communication and cryptography
- Quantum communication networks
- Outlook on Quantum metrology and Quantum imaging
Intended Learning Outcomes
Goals: The course will give a basic introduction into the usage of quantum states of light for the exchange of generation of quantum light and schemes that leverage these states for the exchange of information, ranging from fundamental concepts and experiments to state of the art implementations for secure communication networks. The course will also give an outlook to aspects of Quantum metrology and imaging. After active participation in the course, the students will be familiar with the basic concepts and phenomena of quantum information exchange and some aspects related to the practical implementation thereof. They will be able to apply their knowledge in the assessment and setup of experiments and devices for applications of quantum information processing
-
Quantum Field Theory (Ammon)
Content
- Principles of relativistic quantum field theories
- Quantization of Klein-Gordon , Dirac , and electromagnetic fields
- Perturbation theory and Feynman diagrams
- S matrix and cross sections
- Functional integrals
- Effective effects and correlation functions
- Regularization and renormalization
Intended Learning Outcomes
Teaching the basic principles and structures of quantum field theories. Obtaining abilities to describe the interactions of elementary particles and to calculate important scattering and decay processes.
-
Quantum Imaging and Sensing (Setzpfandt/Pertsch)
Content
- Basic introduction to relevant concepts of quantumoptics
- Generation of photon pairs
- Fundamentals of two-photon interference
- Applications of two-photon interference
- Optical quantum metrology
- Ghost Imaging
- Quantum microscopy
Intended Learning Outcomes
The course will give a basic introduction into the usage of quantum light, in particular photon pairs, for imaging and sensing. To this end, many basic concepts and applications will be introduced and discussed. Furthermore, students will learn how to mathematically describe quantum sensing schemes in order to understand and predict their propreties. After active participation in the course, the students will be familiar with the basic concepts and phenomena of quantum imaging and sensing and will be able to apply the derived formalism to similar problems.
-
Quantum Optics (Setzpfandt)
Content
- Basic introduction to quantum mechanics
- Quantization of the free electromagnetic field
- Non-classical states of light and their statistics
- Experiments in quantum optics
- Semi-classical and fully quantized light-matter interaction
- Non-linear optics
Intended Learning Outcomes
The course will give a basic introduction into the theoretical description of quantized light and quantized light-matter interaction. The derived formalism is then used to examine the properties of quantized light and to understand a number of peculiar quantum optical effects. After active participation in the course, the students will be familiar with the basic concepts and phenomena of quantum optics and will be able to apply the derived formalism to other problems.
-
Theory of Nonlinear Optics (Peschel)
Content
- Types and symmetries of non-linear polarization
- Coupling between optical fields and nonlinear matter
- Solutions of non-linear evolution equations
- Frequency conversion in crystals with quadratic nonlinearity
Intended Learning Outcomes
The course provides the theoretical background to understand nonlinear optics.
Recommended Reading
… on nonlinear materials
- Butcher and Cotter, The Elements of Nonlinear Optics, Cambridge University Press, 1990.
- Richard Lee Sutherland, Handbook of nonlinear optics, 2003.
- Govind Agrawal, Contemporary nonlinear optics, Academic Press 1992.
…on general nonlinear optics
- Schubert and Wilhelmi, Nonlinear optics and quantum electronics, 1986.
- Jerome V. Moloney and Alan C. Newell, Nonlinear optics, 1990.
- Bahaa Saleh and Malvin C. Teich, Fundamentals of Photonics, Wiley, 2007.
-
Internship (individually choosen)
Module Components
Practical course of 300 h. Depending on the topic this total workload should be distributed approximately as: 50 h introduction to the research topic (study of relevant literature, …), 190 h research work (in the lab for experimental topics and atcomputer etc. for theoretical topics), 50 h preparation of the final report, and 10 h preparation and carrying out presentation of the results
Intended Learning Outcomes
Carrying out scientific labwork in photonics together with a research team; preparation of a written scientific report; presentation and defense of the results in an oral presentation.
-
Research Project (individually choosen)
tba
-
2D Materials (Soavi)
Content
- Graphene: electrical and optical properties; Applications in electronic and optoelectronic
- Semiconducting 2D materials: Coulomb screening and the concept of excitons; Optical spectroscopy of excitons; Optoelectronic applications
- Heterostructures: electron and exciton interactions in layered heterostructures
Intended Learning Outcomes
- Mastering the basics and methods of two-dimensional materials
- Ability to work independently on problems in the field of two-dimensional materials
-
Advanced Quantum Field Theory (Ammon)
Content
- Anomalies in quantum field theory
- Quantum field theory at finite temperature and density
- Non-equilibrium dynamics of field theories
- (Quantum) phase transitions
- Introduction to compliant field theory
- Topological objects in quantum field theory
Intended Learning Outcomes
Impart thorough knowledge of advanced methods in quantum field theory.
-
Atomic Physics at High Field Strenghts (Stöhlker)
Content
- Strong field effects on the atomic structure
- Relativistic and QED effects on the structure of heavy ions
- X-ray spectroscopy of high-Z ions
- Application in x-ray astronomy
- Penetration of charged particles through matter
- Particle dynamics in of atoms and ions in strong laser fields
- Relativistic ion-atom and ion-electron collisions
- Fundamental interaction processesScattering, absorption and energy loss
- Detection methods
- Particle creation
Intended Learning Outcomes
The Module provides insight into the basic techniques and concepts in physics related to extreme electromagnetic fields. Their relevance to nowadays applications will be discussed in addition. The Module also introduces the basic interaction processes of high-energy photon and particle beams with matter, including recent developments of high intensity radiation sources, such as free electron lasers and modern particle accelerators. Experimental methods and the related theoretical description will be reviewed in great detail.
-
Atomic Theory (S. Fritzsche)
Content
- Short review of hydrogenic atoms
- Independent-particle model & Hartree-Fock theory
- Interaction with the radiation field
- Correlated many-body theory
- Atomic collision theory
- Basics of the density matrix theory
- Atoms and forces in (intense) light fields
- Laser cooling and trapping; ions traps
- Rotating-wave approximation
Intended Learning Outcomes
Learning the basics of atomic structure and atomic collision processes.
-
Computational Quantum Physics (S. Fritzsche)
Content
- Coulomb problem
- Particles with spin
- Qubits, quantum registers and quantum gates
- Representation of pure and mixed states (Bloch sphere)
- Composite systems, indistinguishable particles
- Hartree-Fock method
- Coupling of angular momenta
Intended Learning Outcomes
- Learning computer algebraic and numerical methods in the description of simple quantum models
- Ability to independently solve simple models and tasks, formulate pseudo-code and deal with computer algebra systems more efficiently
-
Computational Physics III and IV (Brügmann)
Content
Computational Physics III
- Partial Differential Equations
- Fundamentals of differential equations
- Introduction to elliptic, parabolic and hyperbolic differential equations
- Explicit and Implicit procedures, stability analysis
- Poisson equation, diffusion equation, advection equation, wave equation
- Shocks
- Difference method
- Pseudo spectral methods
- Multiple Grids
Computational Physics IV
- Machine Learning in Physics
- Basics of Machine Learning, Neural Networks and Deep Learning
- Sample Applications in Physics, Pattern Recognition, Time Series Analysis, Monte Carlo Methods
Partial Differential Equations:
• Fundamentals of differential equations
• Introduction to elliptic, parabolic and hyperbolic differential equations
• explicit and implicit procedures, stability analysis
• Poisson equation, diffusion equation, advection equation, wave equation,
• shocks;
• difference method,
• pseudo spectral methods,
Partial Differential Equations:
• Fundamentals of differential equations
• Introduction to elliptic, parabolic and hyperbolic differential equations
• explicit and implicit procedures, stability analysis
• Poisson equation, diffusion equation, advection equation, wave equation,
• shocks;
• difference method,
• pseudo spectral methods,
Partial Differential Equations:
• Fundamentals of differential equations
• Introduction to elliptic, parabolic and hyperbolic differential equations
• explicit and implicit procedures, stability analysis
• Poisson equation, diffusion equation, advection equation, wave equation,
• shocks;
• difference method,
• pseudo spectral methods,
Intended Learning Outcomes
Computational Physics III
Mastering the basics and methods of partial differential equations and machine learning in physics
- Mastering the basics and methods of partial differential equations and machine learning in physics
- Ability to work independently on a numerical project
Computational Physics IV
- Mastering the basics and methods of machine learning in physics
- Ability to work independently on a numerical project
-
Experimental nonlinear optics (Paulus)
Content
- Propagation of light in crystals
- Properties of the non-linear susceptibility tensor
- Description of light propagation in non-linear media
- Parametric effects
- Second harmonic generation
- Phase-matching
- Propagation of ultrashort pulses
- High-harmonic generation
- Solitons
Intended Learning Outcomes
This course gives an introduction to optics in non-linear media and discusses the main non-linear effects.
-
Fundamentals of Modern Optics (Pertsch)
Content
- Basic concepts of wave optics
- Dielectric function to describe light-matter interaction
- Propagation of beams and pulses
- Diffraction theory- Elements of Fourier optics
- Polarization of light
- Light in structured media
- Optics in crystals
Intended Learning Outcomes
The course covers the fundamentals of modern optics which are necessary for the understanding of optical phenomena in modern science and technology. The students will acquire a thorough knowledge of the most important concepts of modern optics. At the same time the importance and beauty of optics in nature and in technology will be taught. This will enable students to follow more specialized courses in photonics.
-
Innovation Methods in Physics (Pertsch/Kretzschmar)
Content
- Rapid prototyping technology in photonics
- Innovation management and design thinking
- Hands-on/practical examples of photonics prototyping
- Entrepreneurial skills and business modelling
- Basics of intellectual property rights
Intended Learning Outcomes
The students will learn how the results of their scientific research can be turned into relevant innovations as an important part of their future career. On the one hand, the course will enable students to understand and to drive innovation processes in photonics companies. On the other hand, students will develop an entrepreneurial skill set for the independent economical exploitation of scientific ideas. Therefore, the course introduces the basic knowledge on innovation management, entrepreneurship, and intellectual property rights. To practice their skills, the students will also conduct their own photonics innovation project during the semester by working hands-on in small teams in the photonics makerspace Lichtwerkstatt. During this practical part, they acquire and apply a thorough knowledge of photonic rapid prototyping technology (e.g. 3d- scanning and printing, laser cutting, microcontrollers, ...) and the most important creativity methods and project management skills. To cover this range of topics, the course will be supported by guest lecturers from different sectors (academia, industry).
-
Gauge Theories (Gies)
Content
- Gauge symmetry
- Classical Yang-Mills theory
- Quantization of gauge theories / BRST formalism / Gribov problem
- Perturbation theory
- Semiclassical expansions
- Topological configurations
- Confinement criteria and scenarios
Intended Learning Outcomes
Comprehension of concepts and methods, and acquiring technical skills for the theoretical treatment of gauge theories with applications in particle physics.
-
Integrated Quantum Photonics (Gili/Pertsch)
Content
The lecture will cover a significant part of integrated quantum photonics, which is one of the pillars of the current quantum technology development. In particular, the lecture will cover the following topics
- Integrated optics on a single photon level
- Generation and manipulation of quantum states of light using integrated waveguides
- Overview over integrated photonic platforms and fabrication of passive and active waveguide structures
- Quantum walks in linear and non-linear waveguide lattices
- Introduction to photonic quantum computation and simulation
- Measurements using superconducting nanowire single photon detectors and transition edge sensors
Intended Learning Outcomes
The course should provide the participating students with a profound knowledge on the state of the art of integrated optics used for the realization of quantum optical devices. After active participation in the course, the students will be familiar with the basic concepts and phenomena of integrated quantum photonics and will be able to develop own concepts for integrated quantum circuitry. The intended learning outcome is that the students are introduced to the basics on the field of integrated quantum optics and its applications. Therefore, course starts with an overview on the generation of non-classical states of light with special attention on integrated solutions. Afterwards several integrated photonic platforms will be discussed ranging from fabrication to performance and useability. Based on that the on-chip manipulation of non-classical states of light will be discussed. This starts with the very general concept of quantum walks and continues towards quantum simulation. It ends with an introductory to photonic quantum computing with a clear focus on practical implementation of quantum photonic gate structures. The course closes with the discussion on non-classical light detection in integrated photonics.
-
Introduction to Nanooptics (Pertsch)
Content
- Surface-plasmon-polaritons
- Plasmonics
- Photonic crystals
- Fabrication and optical characterization of nanostructures
- Photonic nanomaterials / metamaterials / metasurfaces
- Optical nanoemitters
- Optical nanoantennas
Inteded Learning Outcomes
The course provides an introduction to the broad research field of nanooptics. The students will learn about different concepts which are applied to control the emission, propagation, and absorption of light at subwavelength spatial dimensions. Furthermore, they will learn how nanostructures can be used to optically interact selectively with nanoscale matter, a capability not achievable with standard diffraction limited microscopy. After successful completion of the course the students should be capable of understanding present problems of the research field and should be able to solve basic problems using advanced literature.
-
Introduction to String Theory and AdS/CFT (Ammon)
Content
Introduction to concepts of string theory and AdS/CFT correspondence, in particular:
- Relativistic bosonic string and its quantization
- Open strings and D-branes
- Aspects of conformal field theory
- Polyakov path integral
- Scattering of strings
- Low energy effective action
- Dualities in string theory
- Compactification scenarios
- Introduction to AdS / CFT
- Main tests of AdS / CFT
- Extension and application of AdS / CFT
Intended Learning Outcomes
Impart thorough knowlege of string theory and AdS/CFT duality.
Impart thorough knowledge of string theory and AdS/CFT duality
Introduction to concepts of string theory and AdS/CFT correspondence, in particular:
• relativistic bosonic string and its quantization
• open strings and D-branes
• aspects of conformal field theory
• Polyakov path integral
• scattering of strings
• low energy effective action
• dualities in string theory
• compactification scenarios
• introduction to AdS / CFT
• main tests of AdS / CFT
• extension and application of AdS / CFT
Introduction to concepts of string theory and AdS/CFT correspondence, in particular:
• relativistic bosonic string and its quantization
• open strings and D-branes
• aspects of conformal field theory
• Polyakov path integral
• scattering of strings
• low energy effective action
• dualities in string theory
• compactification scenarios
• introduction to AdS / CFT
• main tests of AdS / CFT
• extension and application of AdS / CFT
-
Lattice Field Theory (Wipf)
Content
- Path integral for quantum field theories
- Euclidean formulation and quantum field theories in thermal equilibrium
- Lattice field theory as spin models in Statistical Physics
- Rigorous results and approximations
- Stochastic methods, Monte Carlo simulations
- Renormalization group, critical phenomena
- Gauge theories on a space-time grid
- Quantumchromodynamic on a lattice
Intended Learning Outcomes
- The course covers theoretical concepts and methods necessary to understand (discretized) Quantum Field Theories.
- The students will learn stochastical and numerical methods to simulate spin models and lattice field theories.
- They will aquire skills to independently develop numerical algorithms to calculate observables in Elementary Particle Physics, Quantum Field Theory and Statistical Physics.
-
Molecular Quantum Mechanics / Quantum Chemistry II (Gräfe/Croy)
Content
Building on Module PAFMQ100, in-depth and advanced knowledge of advanced methods of theoretical chemistry is taught. This includes (time-dependent) density functional theory as well as an introduction to numerical methods, concepts and algorithms for the description of molecular systems that exchange energy and/or charge with their environment.
Intended Learning Outcomes
Familiarization with advanced methods and concepts, such as DFT/TDDFT. Understanding numerical methods, concepts and algorithms for describing open quantum systems and their application to molecular and nanoscale systems.
-
Nano Engineering (Höppener/Schubert)
Content
- Building with Molecules
- Self-organization and self-assembled coatings
- Chemically sensitive characterization methods
- Nanomaterials for optical applications
- Nanowires and nanoparticles
- Nanomaterials in optoelectronics
- Bottom-up synthesis strategies and nanolithography
- Polymers and self-healing coatings
- Molecular motors
- Controlled polymerization techniques
Intended Learning Outcomes
A large diversity of nanomaterials can be efficiently produced by utilizing chemical synthesis strategies. The wide range of nanomaterials, i.e., nanoparticles, nanotubes, micelles, vesicles, nanostructured phase separated surface layers etc. opens on the one hand versatile possibilities to build functional systems, on the other hand also the large variety of techniques and processes to fabricate such systems is also difficult to overlook. Traditionally the communication in the interdisciplinary field of nanotechnology is difficult, as expertise from different research areas is combined. This course aims on the creation of a common basic level for communication and knowledge of researchers of different research fields and to highlight interdisciplinary approaches which lead to new fabrication strategies. The course includes basic chemical synthesis strategies, molecular self-assembly processes, chemical surface structuring, nanofabrication and surface chemistry to create a pool of knowledge to be able to use molecular building blocks in future research projects.
-
Optical Metrology and Sensing (Staude)
Content
- Basic principles
- Wave optical fundamentals
- Sensors
- Fringe projection, triangulation
- Interferometry and wave front sensing
- Holography
- Speckle methods and OCT
- Phase retrieval
- Metrology of aspheres and freeform surfaces
- Confocal methods
Intended Learning Outcomes
This course covers the main principles of optical measurements and surface metrology.
-
Optoelectronics (Soavi)
Content
- Semiconductors
- Optoelectronic devices
- Photodiodes
- Light emitting diodes
- Semiconductor optical amplifier
Intended Learning Outcomes
In this course the student will learn the fundamentals of semiconductor optical devices such as photodiodes, solar cells, LEDs, laser diodes and semiconductor optical amplifiers.
-
Particles and Fields (Gies)
Content
- Introduction: examples of classical field theories
- Aspects of classical field theory: Lagrange and Hamilton formalism, Noether theorem and charges
- Non-linear scalar field theories: O(N) models, spontaneous symmetry breaking, Goldstone theorem
- Fields / particles as representations of the Lorentz group: classification of representations, spinors, construction of free theories
- Interactive theories: Yukawa models, QED, Abelian Higgs models
- Current aspects of field theories in particle physics
Intended Learning Outcomes
Comprehension of concepts and methods, and acquiring technical skills for the theoretical treatment of field theories with applications in particle physics.
-
Particles in Strong Electromagnetic Fields (Stöhlker)
Content
- Electrons in constant fields
- Electrons in electromagnetic pulses
- Radiation produced by particles in extreme motion
- Radiation reaction
- QED effects in strong laser fields
Intended Learning Outcomes
This course is devoted to the dynamics of charged particles in electromagnetic fields. Starting with motion of electrons in constant magnetic and electric fields, the course continues with the electron motion in electromagnetic pulses (i.e. laser pulses) of high strength (i.e. when laser pressure becomes dominant). Radiation produced by electrons in extreme motion will be calculated for several most important cases: synchrotron radiation, Thomson scattering, undulator radiation. Effects of radiation reaction on electron motion will be discussed. The last part of the course will briefly discuss the QED effects in strong laser fields: stochasticity in radiation reaction, pair production by focused laser pulses and QED cascades. Analytical framework will be complemented with the help of numerical calculations.
-
Physics of the Quantum Vacuum in Strong Fields (Gies)
Content
- Theoretical foundations of quantum electrodynamics (QED) in strong electromagnetic fields
- Derivation of elementary signatures of the strong field QED
- Discussion of proposals for their demonstration with current experimental methods
Intended Learning Outcomes
Imparting concepts and methods and gaining the skills to deal with quantum electrodynamics issues in strong electromagnetic fields.
-
Quantum Information Theory (Gärttner/Sondenheimer)
Content
- Basic introduction to quantum optics
- Quantum light sources
- Encoding
- Transmission and detection of information with quantum light
- Quantum communication and cryptography
- Quantum communication networks
- Outlook on Quantum metrology and Quantum imaging
Contributed lectures by Dr. Sondenheimer
- Open quantum systems, Density matrix formalism, Generalized measurements, Quantum channels
- Superdense coding, quantum teleportation
- Entanglement theory
- Bell inequalities, CHSH inequalities
- Quantum circuits, universal gates
- Quantum error correction
Intended Learning Outcomes
The course will give a basic introduction into the usage of quantum states of light for the exchange of information. It will introduce contemporary methods for the generation of quantum light and schemes that leverage these states for the exchange of information, ranging from fundamental concepts and experiments to state of the art implementations for secure communication networks. The course will also give an outlook to aspects of Quantum metrology and imaging. After active participation in the course, the students will be familiar with the basic concepts and phenomena of quantum information exchange and some aspects related to the practical implementation thereof. They will be able to apply their knowledge in the assessment and setup of experiments and devices for applications of quantuminformation processing.
-
Solid State Optics II (H. Schmidt)
tba
-
Structure of Matter (Stenzel)
Content
- Classical interaction of light with matter
- Basic knowledge on quantum mechanics
- Einstein coefficients and Plancks formula
- Selection rules
- Hydrogen atom and helium atom
- Introduction to molecular spectroscopy
- Dielectric function and linear optical constants
- Kramers-Kronig-Relations
- Linear optical properties of crystalline and amorphous solids
- Basic nonlinear optical effects
Intended Learning Outcomes
The course is an introduction to the principles of the optical response of materials.
-
The Standard Model of Particle Physics (Wipf)
Content
Overview of the standard model of particle physics including:
- Symmetries, quantum electrodynamics
- Strong interaction
- The quark model and quantum chromodynamics
- Hadrons and asymptotic freedom
- Weak interactions and the Higgs effect
- Scattering experiments
- Limits of the Standard Model
Intended Learning Outcomes
Impart thorough knowledge of particle physics phenomenology and its fundamental concepts.
-
Theoretical Solid State Physics (Peschel)
Content
- Crystal structures and elastic properties of solids
- Electronic properties of crystals
- Approximate methods for electronic band structure
- Semiconductors and defect physics
- P-n junctions
- Microscopic description of charge transport
- Properties of alloys
- Nanostructures and interfaces
- Optical and dielectric properties of solids
- Magnetism and superconductivity
Intended Learning Outcomes
The course covers advanced topics of solid state physics, with a specific focus on the theoretical understanding of the properties of electrons in crystals. An effort is made to remain as rigorous as possible in the theoretical and mathematical treatment, while keeping the presentation at an accessible level through the presentation of interesting applications to experiments and advanced technology. After completion of the course the students will master the relation between electronic structure of crystalline solids and their dielectric, optical, transport, magnetic properties.
-
Symmetries in Physics (Wipf)
Content
- Symmetries and groups
- Space and space-time symmetries
- Conserved currents and charges
- Discrete groups and continuous Lie-groups
- Representations of groups, theory of characters, reductions of representation
- Invariant integration on Lie-Groups
- Lie-algebras and their representations
- Classification of semi-simple Lie-algebras
- Selected application of group theory and representation theory in solid state physics, atomic and molecular physics, quantum field theory and particle physics
Intended Learning Outcomes
The course covers theoretical concepts of discrete and continuous groups, Lie-algebras and their representations with relevant applications in physics. The students will learn how to exploit symmetry principles to simplify or even solve problems in all branches of physics where symmetry principles play a role.
-
Supersymmetry (Wipf)
Content
- Supersymmetric quantum mechanics
- Symmetries and spinors
- Wess Zumino models
- Supersymmetry algebra and representations
- Superspace and superfields
- Supersymmetric Yang-Mills theories
Intended Learning Outcomes
The students will learn the structure and properties of supersymmetric theories and the basics for understanding developments in particle physics and string theory. They will aquire skills to calculate simple processes in supersymmetric theories.
-
Topics of Current Research: Quantum Field Theory (Ammon)
Content
- Further, in-depth topics in the field of quantum field theory
- Topics from current areas of research
Intended Learning Outcomes
- Specialisation in a special field of quantum field theory
- Independent handling of exercises
- Ability of literature review
-
Topics of Current Research: Gravitational Theory (Brügmann)
Content
- Further, in-depth topics in the field of gravitation theory
- Topics from current areas of research
Intended Learning Outcomes
- Specialization in the special field of gravitation theory
- Independent handling of exercises
- Ability of literature review
-
Master degree thesis (individually choosen)
tba